I was talking to her on the phone and said, "Oh, I emailed you a photo of Cervelo's wind tunnel together with my thoughts about it." Her response was "You know about wind tunnels too?"
It turns out that when you study aeronautics, you can't help but learn about wind tunnels. The physics of what actually goes on when an airplane flies are pretty much the same physics going on inside a wind tunnel. And in some ways it is a lot easier to look at the fixed and symmetric venturi of a wind tunnel than the comparatively complex environment of an airplane flying through the annoyingly free sprited atmosphere. But for all that, wind tunnels remain interesting and quite complex creations -- at least once the desired level of precision and control reaches beyond that of rough approximations and undergraduate aeronautics classes.
So what does make a wind tunnel so interesting? It is the very task of simulating, in a very controlled and in many ways deeply unrealistic environment, a very complex situation. Let's start with this: intuitively, using only stuff sitting around at home, how would you build a wind tunnel. Probably like this, the way I used to as a child (really):
Hold your test subject (Mk.1 Paper Aviation Device) so that a fan blows air over it. Measure the lift and drag forces on Mk.1 PAD and plot on graph paper. A nice start, but with one grave error: your position relative to the fan.
It turns out that the air coming off of a fan (or as we call them in wind tunnels, a compressor) is quite turbulent and prone to taking on a swirling action imparted by the spinning blades. Far more effective, then, is to put your model upstream of the blades so that the air is pulled across it rather than pushed. Now since we'd like to have some more control over that environment, encase the model in a box with a few holes for observation and balance beams to take measurements with. This area now gets the fancy name of test section.
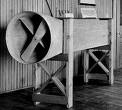
This is pretty good for rough approximations and low speeds. A good first improvement is to put a screen of some kind at the inlet side and between the test section and the compressor. These further help smooth the airflow. Make sure that your test object (wing section, large building, cyclist) doesn't get too close to the walls of the test section so you don't get interference between the flow along the walls (which is disturbed and turbulent) and the flow over the model (which must mimic the smooth flow of air in free space). An interesting exception to this problem occurs when testing objects (cars, bicycles) that operate close to the ground. Here you want to simulate the effect of the ground, but it turns out that since, for a moving vehicle, the ground and the airmass and the vehicle all have different relative velocities. As a result, some of the amazing wind tunnels in use by Formula 1 teams actually have "rolling floors" -- giant belts that roll along under the model to simulate the aerodynamic issues caused by the ground more accurately.
Anyway, back to our model. So far, so good. We're doing quite nicely as long as we're comfortable with atmospheric temperatures and pressures and don't try to go too fast. Since airplanes cruise at all kinds of confusingly complex altitudes (where it can also get really, really cold) it would be nice to somehow simulate those conditions. It also turns out that taking a bunch of air, sucking it with giant fans (er, I mean compressors) and dumping it back out can anger the neighbors who live downstream of you and who may not like sudden man-made windstorms and all the resulting noise. It can also be quite a bit more efficient to loop the air back around, so that the exhaust of the compressors flows around in a big loop and actually feeds back into the front of the test section.
I know, we don't want to blow on our model, but suck on it. Thank you, Lewis Black. But we aren't really blowing -- the suction side of the compressor is much closer to the test section than the blowing side. And we have a huge loop full of guide veins and settling chambers (fat areas in our recirculating tube that serve to damp out turbulence) and screens and whatnot.
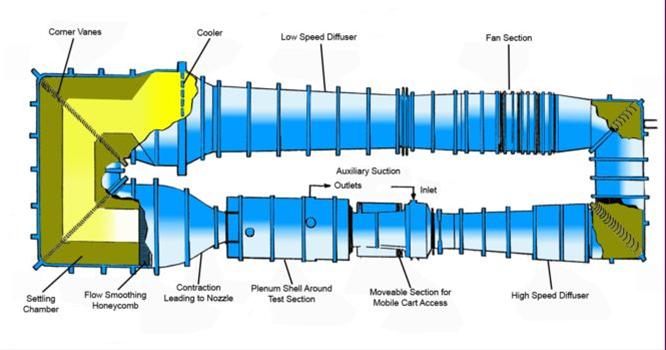
The problem is one of scale. Pretend you are a molecule of Oxygen or Nitrogen or some other common atmospheric constituent. You're a piece of air, don't sweat the details. You're sitting there, floating along happily, when along comes a wing, swooshing by and yanking you out of your happy daze as it extracts some of that magic property of lift. If that wing belongs to a bumble bee, it seems to flash by, larger than you, but not so enormously huge as all that. On the other hand, if you are up there in the tropopause and a 787 comes flying buy (in addition to you apparently being a few months in the future) its wing is moving much faster but is comparatively enormous. It flashes by for an eternity, like watching a city sweep by, thousands of times longer than the bumble bee wing.
And there you have it. Though the actual mechanics have more to do with the relationship between viscous and inertial forces, the basic "deal" of the reynolds number is that of scale. Compared to the bits of air through which they pass, a bumble bee wing is tiny and a 787 wing is huge. The difference is profound, since at the scale of an insect wing, air is much more dramatically viscous than anything we can imagine.

Testing airplanes at 600 mph requires a lot more energy -- an impossible amount of energy for a wind tunnel large enough to test a commercial jet. So scale model testing is necessary. And we now have the opposite problem of our "unflyable" bumble bee. Scale the airplane to 1/50th of its size and suddenly the air is way to sparse. The solution is to increase the density of the molecules of air -- pack them closer together so that they scale to match the environment expected at cruise speed and cruise altitude.
The two approaches to this are pressurization and refrigeration. The former is obvious -- it just plane squishes more and more and more molecules of air into the space around the model. But that only goes so far before engineering gets in the way and our wind tunnel wants to explode. The other approach is to chill the working fluid. All substances (save for some aberrant behavior right around phase changes) increase in density as they are chilled. By using both conditions, modern wind tunnels can produce conditions very close to (and sometimes identical to) that experienced by an aircraft in flight.
Ironically, the process of accelerating the air requires tens of thousands of horsepower -- and dumps a lot of waste heat into the wind tunnel. So cooling the tunnel and its contents is an uphill struggle. You can imagine how much electricity it takes to spin 12,000hp motors, remove the waste heat they produce, and chill the air to 100 degrees below zero...

As a result, wind tunnels today are used primarily as validation tools. Models are tested simultaneously in a tunnel and using CFD (computational fluid dynamics) code. Engineers use the results of these selected comparison runs to tweak their code until the results agree perfectly. The design is then roughed out using the CFD simulations (which require prodigious computing power -- but that's cheap these days) to produce a few candidates. These candidates are run through the wind tunnel and the results of the tunnel runs and CFD simulations are analyzed together to select a final approach. Usually the CFD simulations will look at all kinds of variations and the wind tunnel only at a few crucial cases.
This cycle of CFD approximation leading to wind tunnel verification proceeds until the design is finished. As CFD has improved, the time spent in the tunnel should have decreased, but the increasing drive for optimization means that engineers still find it worth their while to run tests in the tunnel. CFD codes were once crude and are improving, but with billions of dollars on the line, a little due diligence is still the way to go.
For dramatically unpredictable environments -- like a cyclist with jersey billowing, cross wind blowing, wheels spinning, peloton careening -- CFD is actually just about impossible. Even if gifted with the supercomputing power of Boeing's Crays, an aero obsessed cycle company like Cervelo couldn't accurately test in a purely virtual environment. Instead they use computers to design naked components such as frame cross sections, but rely on the wind tunnel for the synthesis of the parts into the whole. Since the test situations are easily atmospheric, the tunnel needn't be complex, either, and the whole situation is a lot more fun when you've got some dude in a Team CSC jersey pedaling away in your test section.
![B4BF1790[2].jpg](http://lh4.ggpht.com/strauss.nick/SH6qmB1BajI/AAAAAAAAAIg/rUT0apXpcLw/B4BF1790%5B2%5D.jpg?imgmax=800)
Note, by the way, the turntable for testing in crosswinds. Also note that moving floor technology hasn't quite made it to cycling yet. Without the ground hugging aerodynamic downforce of Formula 1 cars, there may not be a need. And, without the $150 million a year budgets, there may not be the cash!
1 comment:
I am glad you took the time and wrote this.
Carmella
Post a Comment